From PopularMechanics by David Hambling
GPS is a world-changing technology. It’s also incredibly fragile, easily spoofable, and consistently hackable.
That’s why the U.S. Navy and Marine Corps are looking to the stars for a navigational Plan B.
Twenty-five years ago, in 1995, the U.S. Air Force reached a milestone: Its satellite Global Positioning System became fully operational.Today, GPS directs our world, from supertankers to pizza deliveries. But before the creation of this modern navigational marvel, humans looked to the heavens to find their way.
For centuries, using a sextant, a sailor could mark a ship’s location by making a few calculations to determine a star’s position relative to the horizon.
Now a high-tech version of this maritime tradition is finding its way back into practice.
GPS is indispensable these days—but it’s still incredibly fragile.
It can be spoofed with a fake satellite signal, hacked by an adversary, or simply destroyed. Spoofing, hacking, or destroying the stars? Not happening.
GPS may have revolutionized the way we navigate, but for years celestial navigation has been undergoing a quiet revolution of its own.
DON’T CALL IT A COMEBACK
The U.S. Navy became less reliant on the traditional sextant back in WWII with the advent of radio navigation tools, but the heavens have remained an important map for sailors and especially pilots. WWII bombers like the B-17 Flying Fortress had an ‘astrodome,’ a transparent hemisphere that allowed the navigator to take star sightings.
The astrodome, found on the nose of the B-17G in front of the cockpit window, helped navigators find their way using the stars.
SMITHSONIAN INSTITUTIONWIKIMEDIA COMMONS
After the war and the birth of the rocket engine, scientists set about giving missiles the same kind of celestial smarts.
The SM-62 Snark cruise missile, developed in the 1950s, featured the first automated celestial navigation system, a mechanical-electrical device that weighed almost a ton.
The SM-62 Snark cruise missile, developed in the 1950s, featured the first automated celestial navigation system, a mechanical-electrical device that weighed almost a ton.
The Snark’s navigator had a small telescope mounted on three gimbals that rotated to point it to a given area of the sky.
An early photosensor located the stars in the field of view and measured their angles, identifying them from a star catalog.
The makers claimed that the Snark was able to navigate to within about three kilometers, but it never worked that well in practice.
Then again, you don’t need much navigation finesse when you’re delivering a 3.8-megaton nuclear warhead.
Then again, you don’t need much navigation finesse when you’re delivering a 3.8-megaton nuclear warhead.
How a Sextant Works
Getty Images
It may look daunting, but a sextant simply measures the angle between a heavenly body—star, planet, moon, or sun—and the horizon.
Key components of a sextant are a miniature telescope, a half-silvered mirror (through which the telescope looks), and an index mirror on a moving arm.
Looking at a celestial body through the telescope, you rotate the index mirror until it appears to be on the horizon.
You then note the angle of the arm that corresponds to the angle of the celestial body from the horizon.
Measuring the angle from one heavenly body tells navigators that they are somewhere on a circle on the Earth’s surface, known as the position circle.
For example, at any given moment there are a range of positions from which the sun is at exactly 35 degrees, lying on a circle about 3,000 miles in diameter.
Navigators can plot this circle on the map if they have the relevant charts and know the exact time.
Because the circle is so large, it appears as a straight line on a local map.
Plotting two such circles for two celestial objects gives two lines, and where they intersect is your location—in theory.
Careful navigators usually take three or more sightings, because the lines do not necessarily meet at a single point.
Three lines enclose a triangular area of possible positions traditionally known as a ‘cocked hat.'
Decades later, engineers built modernized celestial navigators, now about the size of a microwave oven, into the B-2 bomber and Trident missile.
An observatory at Boeing’s Guidance Repair Center in Heath, Ohio, checks the location of the North Star every few days, and uses the measurements to calibrate military navigation and guidance systems. In modern missiles, celestial guidance is used in conjunction with an inertial navigation system (INS).
While celestial navigation survived in niche applications like missiles, GPS has edged out the competition because it’s accurate and cheap.
But GPS works only when there’s a good satellite signal, and adversaries are very much aware of that weakness.
GPS: REVOLUTIONARY…WHEN IT WORKS
In 2018, Russian GPS jamming disrupted a NATO exercise in Scandinavia, and Chinese GPS spoofing signals can cause ships in many coastal areas to see false locations.
Modern military GPS systems feature a range of electronic guards to prevent jamming and spoofing, including directional antennas and a special M-code military satellite signal.
Even with these safeguards, a GPS signal may simply not be there: There are concerns about whether the satellite system might be hacked, or the satellites themselves knocked out by an enemy or a massive solar storm.
This has focused military minds on alternatives.
A soldier checks a map during NATO’s Trident Juncture exercise in
October 2018.
Participants accused the Russian government of GPS jamming
during the exercise.
LEON NEALGETTY IMAGES
The Navy’s Automated Celestial Navigation System would replace manual shipboard measurements with something more accurate, while Special Operations Command experts are developing a handheld device for commandos.
Both pieces of tech are aiming for GPS-level precision.
“The best accuracy for celestial navigation with certainty is within a couple of meters,” says Benjamin Lane of the Advanced Position, Navigation & Timing Instrumentation unit at Draper Laboratory in Cambridge, Massachusetts.
“In practice, we are within a factor of a few of that.”
SPOOFING, HACKING, OR DESTROYING THE STARS? NOT HAPPENING.
The new systems use infrared rather than visible light for locating stars, allowing daytime navigation.
The stars shine just as brightly in the day sky as they do at night, but their light is masked by sunlight scattered by the atmosphere.
The scattering is strongest at short wavelengths.
If you’ve ever glimpsed the sky, you know that blue light is scattered the most.
But glimpse that same sky with a filter that allows only infrared light, and the sky suddenly becomes dark—and filled with stars.
“Twenty years ago, these infrared sensors were quite expensive,” says George Kaplan, a consultant for the U.S. Naval Observatory.
“Twenty years ago, these infrared sensors were quite expensive,” says George Kaplan, a consultant for the U.S. Naval Observatory.
“Since then, costs have been going down and the pixel count is getting higher.”
The rise of celestial navigation is also helped along with another piece of tech called a “phased optical array,” which does not need to be pointed at a section of the sky like a telescope does.
The rise of celestial navigation is also helped along with another piece of tech called a “phased optical array,” which does not need to be pointed at a section of the sky like a telescope does.
This type of sensor does not have a lens to focus, but instead has an array of tiny meta-material antennas to capture light.
A processing unit can phase-shift the signal from each antenna to achieve the same effect as a movable, focusing lens.
Lane and his team have already demonstrated a working prototype of such a sensor.
A view of SpaceX’s Starlink constellation at night.
YURI SMITYUK / GETTY IMAGES
TWINKLE, TWINKLE, LITTLE SATELLITE
But the biggest development, being pursued by both Draper Laboratories and Trex Enterprises, is an approach that overcomes one of the biggest limits to accuracy.
Traditional celestial navigation requires measuring the angle of a star or planet in the sky.
To do this you need to precisely locate the horizon or, alternatively, the azimuth or true vertical.
That sounds simple, but it isn't.
“Trex has tried infrared detection of the horizon,” says Kaplan.
“The problem is that the horizon is refractive; it’s very sensitive to temperature differences. You get all sorts of odd reflections, including mirage.”
Others have sensed the azimuth by the polarization of the sky, or by the refraction of starlight, or even angular motion sensors detecting the Earth’s rotation.
None of them comes close to GPS accuracy. But this new approach takes readings from human-made satellites rather than stars, sidestepping the horizon altogether.
A Military History of Celestial Navigation
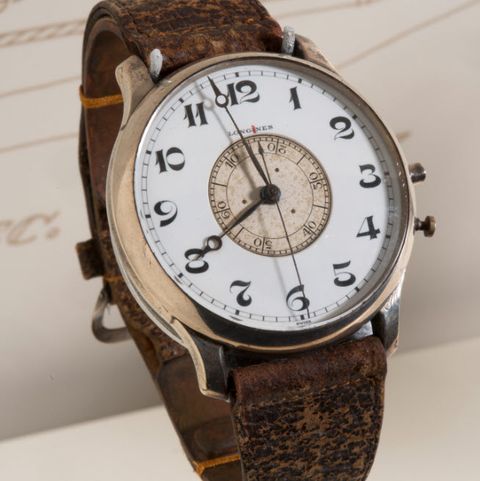
Longines Weems A-11 Aviators Watch
The precise time is vital for celestial navigation: an uncertainty of 30 seconds means a distance error of ten kilometers.
The precise time is vital for celestial navigation: an uncertainty of 30 seconds means a distance error of ten kilometers.
The Dutch aviator Capt. PVH Weems developed a watch with a second hand that could be set exactly to radio time signal broadcasts, which the U.S. Navy started in 1904, reducing errors
The U.S. Army Air Corps commissioned its own version of the watch in 1937, the A-11, and issued one to every new aviator.
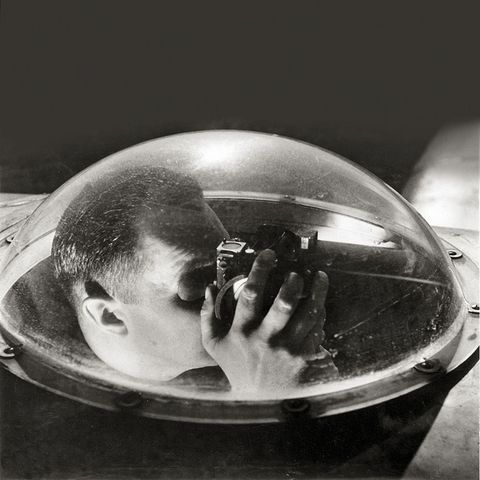
Plexiglas Astrodome
Introduced just in time for WWII bombers, the astrodome was a wonder of materials technology: Plexiglas, a rugged transparent plastic, formed into a dome giving a clear 360-degree view of the night sky.
The astrodome included a hanging point for a sextant so navigators would not have to hold the weighty instrument.
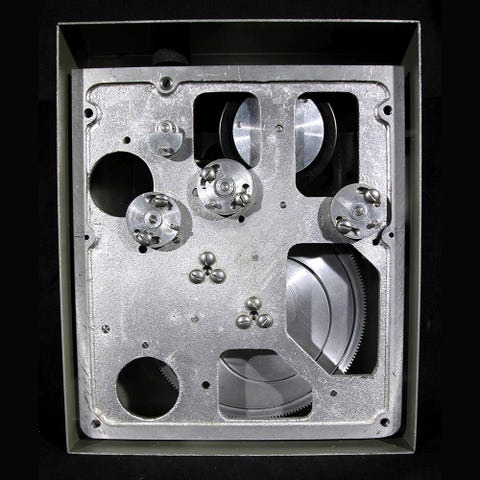
Fairchild-Maxson Mark I Line of Position Computer
Calculating position from star readings was a time-consuming and demanding task—there was no ‘app for that’ in pre-electronic days.
The USAAF automated the process with this 1938 mechanical calculating device.
The navigator dialed in the angle readings and the time, and the 20-pound computer did the rest. Several dozen were built and used by the Air Corps and the Navy, but the design proved too expensive.
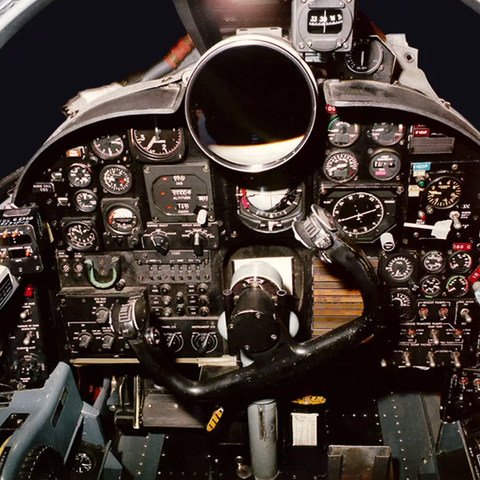
U-2 Driftsight
The dashboard of the U-2 spy plane was dominated by the driftsight, a downward-looking periscope to follow roads and other landmarks for navigation.
When the ground was invisible, flipping a mirror converted the driftsight into a sextant with a view of the sky through a glass bubble on the plane’s nose.
This was helpful in areas like the Arctic with no terrain features and where magnetic compasses were useless.
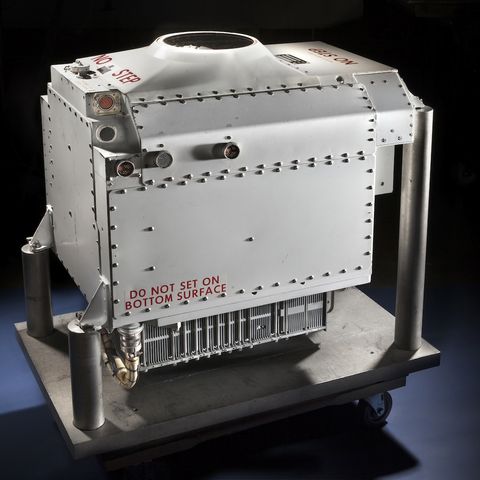
Nortronics NAS-14V2 Navigation System
Affectionately known as R2-D2, the celestial navigator in the SR-71 Blackbird occupied a position immediately behind the pilot.
Measuring 48 x 46 x 31 in. and weighing 300 pounds, it performed the vital function of keeping the Blackbird on track even at Mach 3, ensuring that the plane’s cameras photographed the right area of interest in the ground.
It also helped keep the valuable plane on the safe side of sensitive international borders.
Because satellites are relatively close to the Earth’s surface, observers can calculate their own position based on the angles among different satellites.
The navigator does not need to see the horizon or know the local vertical. Instead, it’s a version of triangulation that any hiker uses when taking angle readings from two mountaintops.
Any visible satellite can be used to navigate if its position is known, and there are now plenty to choose from.
In 2010, fewer than 1,000 satellites were in orbit; that number has more than doubled and is rising fast with mass launches of small satellites. SpaceX’s Starlink constellation alone will add more than 7,000 in the next few years, so there should always be one handy to take a reading.
“Starlink’s satellites are being made less reflective but still trackable,” says Lane.
“If they are predictable, then we can track and use them.”
The only limit is how accurately a satellite’s position is known.
While the orbital track is usually known exactly, a satellite’s position along the orbital track is affected by atmospheric drag, resulting in a deviation of arc minutes (an error of one nautical mile, or 1,952 meters.)
Fortunately, the North American Aerospace Defense Command (NORAD) tracks many of them with high-precision radar, and can supply the data to military users.
Once these celestial navigation tools are ready for combat, they’ll come in different shapes and sizes.
The B-21 Raider bomber will likely have a more compact, highly precise version of the B-2 Spirit’s R2-D2-like unit that sits behind the pilot.
On Navy ships, the Automated Celestial Navigation System will replace the old brass sextants and provide data that can be fed into shipboard computers along with other navigational sensors. Future ballistic missiles will also include advanced celestial navigation.
And with a new handheld device being developed by Special Operations Command, even the humble foot soldier could be steering by the stars.
CELESTIAL LIMITATIONS
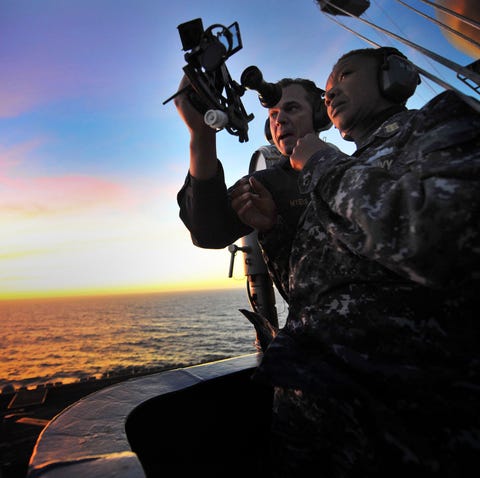
Senior Chief Petty Officer Jonathan Myers teaches Command Master Chief April Beldo how to use a marine sextant on the USS Carl Vinson.
TRAVIS K. MENDOZA
TRAVIS K. MENDOZA
While it is already possible to get a location within 20 meters or so, and devices with more accuracy are in the pipeline, celestial navigation is not quite as handy as GPS.
For one thing, it currently takes much longer to get a reading, often tens of seconds.
For another, Kaplan says that even though infrared imagers work through haze and light cloud cover, they cannot see through dense, low clouds.
For another, Kaplan says that even though infrared imagers work through haze and light cloud cover, they cannot see through dense, low clouds.
Unlike GPS, celestial navigation will not be available 24/7, so it would have to work with an inertial navigation system.
Luckily, these are getting much smaller and cheaper too.
“Another ‘gotcha’ using LEO satellites is that in daytime the light is coming from above,” says Kaplan.
“Another ‘gotcha’ using LEO satellites is that in daytime the light is coming from above,” says Kaplan.
This means the brightest part, the solar array, is pointed away from the Earth and the satellite is much less bright than it is at night.
But the developers are confident that they can overcome these issues, unlocking celestial navigation’s true potential as a GPS substitute—without all the dedicated space hardware, risks of jamming, or vulnerability to cyberattack.
In 1980, the first portable Rockwell GPS receiver was a backpack unit that weighed 20 pounds.
In 1980, the first portable Rockwell GPS receiver was a backpack unit that weighed 20 pounds.
It took five minutes to get a fix and cost around $30,000.
Modern GPS receivers are chip-sized, give an almost instant readout, and cost less than $50.
In 40 years, who knows where the stars might take us.
In 40 years, who knows where the stars might take us.
Links :
No comments:
Post a Comment