From Nautil.us by Charles Digges The internet is a series of tubes.
In the ocean.In late December of 2021, the seafloor near the tiny South Pacific Island nation of Tonga began to rumble.
The restive Hunga Tonga–Hunga Haʻapai volcano was waking up.
In the wee hours of January 15, after days of tremors, the bottom of the ocean finally cracked, disgorging the largest explosion on record.
Four blasts of molten rock that packed 1 billion tons of force each sent a plume 36 miles into the sky.
The blast was so powerful it could be heard in Alaska, 6,000 miles away.
For days afterward, lashed by tsunamis and clouded beneath volcanic ash, the Tongans were unable to call for help.
Severed in the eruption was the
single undersea telecommunications cable that could carry Tongan voices and emails the 514 miles to Fiji, and from there, to the rest of the world.
It was as if a drunken god had tripped over the power cable to the collective computer.
Screens went dark, phones went silent, and the internet disappeared.
The Tongans were all alone.
“We were totally blank from the internet world for at least three days,” said Samisi Panuve, head of Tonga Cable, the company that owns the nation’s subsea connection.
In fact, Panuve said, it would take weeks of exacting repair work at sea aboard highly specialized ships for the line to be fully restored.
All the electrons of information stored on the internet’s servers may only weigh as much as an apple.
So much of the cable was damaged that portions of it had to be remanufactured from scratch.
In the interim, contemporary staples such as Google, Facebook, Amazon, and Netflix—to say nothing of telephone calls and text messages—were only flickeringly available via vexingly slow backup satellites.
Even now, internet coverage on the island can be a little spotty.
It’s still nearly impossible, for instance, to reach the
website for the Tonga Cable Company itself, at least from where I sit in the United States.
For so long, the online world has been so available that its heavenly omnipresence is simply assumed.
We pick up a smartphone or open our laptops, and our consciousness is seamlessly transported to wherever—be it the ear of your grandmother in Budapest, a hotel reservation site in Jakarta, or an office meeting in Oslo—all at almost the speed of light.
But the experience of the Tongans exposes that apparent ubiquity as something a little more precarious.
The online world doesn’t simply rain down from The Cloud: It is transported by something far more tangible, far removed from the weightless data swirls that appear to emanate from Silicon Valley.
All the electrons of information stored on the internet’s servers may only weigh as much as
an apple—but it takes
a couple million pounds of wire to get them to your screens.
For the internet to be the truly global service that it is, many of these wires—most of them no thicker than a garden hose—are sunk full fathom five across the bottom of the ocean, where they lay alarmingly vulnerable to fishing nets, ship anchors, currents, shark bites, scuba divers with saws, earthquakes, and, of course, volcanoes.
These slender strands of mega-charged fiberoptic cables moving terabits per second account for
95 percent of all international data and voice transfers—volumes that blow satellites out of the sky.
What is more shocking than having the vast bulk of non-physical human interaction carried by something that looks like it comes from the lawn care section of a hardware store, is how comparatively rare disconnection calamities like the one that befell Tonga really are.
According to the folks who lay them and fix them, the 870,000 miles of submarine cables invisibly meshing the world together under each of our planet’s oceans demand only about 100 repairs per year—far fewer than their wind- and rain-swept terrestrial cousins.
The telecommunications analytics agency TeleGeography maintains an addictive
map of all the world’s undersea cables, which bears a stylistic—and almost metaphoric—resemblance to the
map of the London Underground.
To access thatmap, which is stored on a server in London, my web requests may follow a few possible routes, according to TeleGeography’s map.
It might leave the continental U.S. through Brookhaven, New York on a cable called
Atlantic Crossing-1, surfacing again at Whitesands Bay on the Irish Sea.
Or it might disembark via Island Park on Long Island traversing the newer
FLAG Atlantic -1cable to the town of Skewjack on the bony finger of England’s Cornwall coast.
These circuits were on my mind as I spoke with Dieter Dillard in France.
Dillard is one of those affable engineers whose enthusiasm for his craft makes it possible for him to explain it to anyone.
He started in the cable business aboard a cable laying ship in the Mediterranean, and over a couple of decades, he worked his way up to CEO of Orange Marine, a company that has laid 164,000 miles—close to 18 percent—of the world’s operational subsea cables.
He’s also whom you call when one breaks—and although Orange Marine wasn’t the company that restored Tonga’s cable (New Jersey-based Subcom was), Dillard knows exactly how it was done.
“The cable laying industry is a small one, and we all know each other,” he told me.
DIGITAL SPIRAL: Looking a bit like a garden hose, a glass-filled cable that can transmit data at nearly the speed of light gets slowly wound onto a ship that will lay it carefully along the ocean floor.
This ship can carry nearly 5,000 miles of cable, enough to cross the Pacific.
Photo courtesy of Orange Marine.He came through crisp and clear over a Microsoft Teams video meeting, the 5,000 or so miles of cable between me in New Orleans and him in Paris amounting to only the slightest little visual flickers.
I imagined, with no easy way of knowing, that our conversation was piped through the
Dunant cable, running from Saint Hilaire-de-Riez on France’s Atlantic Coast to Virginia Beach stateside.
As that’s one of the newer transatlantic cables, laid in 2021, we agreed it was a pretty good guess.
But the sheer number of routes running between the U.S. Atlantic Coast and Europe meant we couldn’t be positive.
And therein, he told me, lies one of the chief protections of our transoceanic communications: redundancy.
Although the companies and telecommunications consortia that own the cables are in competition with one another, Dillard said that each nonetheless rents capacity to others as insurance against a dead line.
If, for example, the company BW Digital’s Hawaiki cable is having trouble updating your Instagram from a beach in New Zealand, your attempts to inspire FOMO might instead travel to the U.S. server via the Southern Cross Cable owned by the eponymous Southern Cross Cable Network—or a dozen other circuitous routes owned by various telecom companies.
And this is, of course, what doomed Tonga: its
single, lonely cable tethering it to the rest of the globe, which was laid in 2013.
Michael Clare, who studies how undersea environments interact with subsea infrastructure at Britain’s National Oceanography Center, told me that the violence of the Hunga Tonga eruption surprised everyone.
A wall of subsea debris was hurtled more than 60 miles across the seafloor toward Tonga’s cable,
according to a paper Clare co-authored.
Such force, though, suggests even a redundant system along a similar route might not have kept the Tongans online in their hour of need.
The first truly intercontinental subsea cable was laid in 1866 between Newfoundland and Ireland by the SS Great Eastern, the biggest ship of its day.
Made of seven copper coils insulated in gutta-percha—a rubbery substance from the tropical sapodilla tree that’s also used to fill modern-day root canals—this cable established a 2,226-mile telegraph link, run by the Atlantic Telegraph Company.
Today, of course, we would consider it intolerably slow.
The first official message it carried was a 98-word dispatch of congratulations from Queen Victoria to President James Buchanan that took 16 hours to arrive.
Another problem was that as electricity traveled the copper, it lost power and began to smear and slur the dots and dashes of its Morse Code vocabulary, making life miserable for the telegraphists.
And that cable didn’t last long.
Attempting to sober up the cable’s diction, the company’s strident head engineer Wildman Whitehouse—a loathed figure in cable laying lore—simply shot more electricity through it, thus baking the insulation and frying the line.
It would be the invention, a few years later, of the
mirror galvanometer by Whitehouse’s more brilliant workplace nemesis, William Thomson, that allowed for the much more precise articulation of electrical pulses that finally put Europe and the U.S. into regular real-time conversation.
For saving the day, Queen Victoria elevated Thomson to “Lord Kelvin”—yes, the Kelvin who invented absolute zero—and he went on to make a fabulous fortune.
By 1956, the advent of much higher capacity coaxial cable began replacing the copper subsea lines.
This enabled crisper transoceanic telephone calls, which, since the late 1920s had only been haltingly possible by radio and only during good weather.
In 1988, fiberoptic cable arrived.
These cables work by sending light pulses down long tendrils of glass, and they made everything that came before obsolete.
The first transoceanic cable message was a 98-word dispatch that took 16 hours to arrive.
The newer cables, like the old, follow 19th-century trade routes—which, in turn, follow the older pathways of human interaction, migration and, in many cases, domination.
On the TeleGeography map, it’s clear that South America and Australia don’t seem to have much to say to each other.
But among the first subsea cables installed were ones linking telegraph operators in England with colonial colleagues in India and Australia.
The new
Amitié cable, a Microsoft-Meta joint venture, moves 400 terabits a second of data between the U.S. and the United Kingdom along one of humanity’s most traversed sea routes.
Meta’s planned
Anjana cable, scheduled to be operational later this year, could be seen to chart Columbus’ route from Spain to the New World.
The business of getting a cable from point A to point B begins on special surveying ships running a chorus of acoustical sounding equipment.
Sonar readings are fed into a bank of shipboard computers that provide remarkably high resolution, allowing engineers to see whether the seabed is sand or hard rock.
These data trace a corridor to pass the cable though as close as possible to the intended route.
The width of the corridor is usually two- or three-times the depth at which the cable will lie, which allows for some—but not much—wiggle room to skirt undersea formations—like cliffs and trenches.
When a survey ship encounters an impassible barrier—a sharp drop not accounted for on nautical charts, for instance—all it can do is back up and attempt to rechart another route as close to the planned route as possible.
With all this high-tech undersea cartography, how did the poor Tongans end up with a cable running so close to a volcano?Clare pointed out that two unavoidable factors beset its route.
First, the Tonga volcano eruption was one of those once-in-a-thousand-years type scenarios.
Given that the expected lifespan of any given cable is about 25 years, he told me, the gamble seemed worth it.
Second, subsea cables are really expensive.
At north of
$80,000 per mile, survey ships get points for sticking as close as possible to the route drawn out by the folks on dry land.
But volcanoes, Clare said, continue to be blind spots, especially in the South Pacific.
When it comes to physically laying cables across the floor of the ocean, Dillard says that the crew of the SS Great Eastern in the 1860s would recognize what crews on Orange Marine vessels are up to today.
“It’s all cable and hooks,” he tells me.
“The mechanics of the process have remained pretty much the same.”
The process of laying underwater cable begins by coiling several thousand miles—and tons—worth of cable onto the specialized circular basins aboard, say, Orange Marine’s vessel the Rene Descartes.
As the cable is fed aboard at port, one person on the ship will walk the cable in a circle, as if coiling the world’s longest garden hose, while other crewmembers literally lie down on it to ensure it doesn’t snag or knot or gain tension.
Upward the cable will coil on top of itself, like a snake in a charmer’s basket until the basin is full.
Even with teams of dozens of people working around the clock, it takes as long as four weeks to load the ship.
The Rene Descartes, which measures about 475 feet long, can pack nearly 5,000 miles of cable—enough to lay an uninterrupted line across the Pacific.
At sea, paying out the cable from deck to seafloor is slow business—and that’s basically all down to managing slack.
Figuring out how much slack to pack is a headache, Dillard says.
Bring too much, and your cable will lie lazily across the seafloor in an unmappable mess; too little, and it could hover like a tightwire between rises on the seafloor, apt to get snagged by surface ships, unable to reach its landing station.
REMOTE WORK: When undersea internet cables break, technicians pinpoint the damage, and other workers deploy an ROV—navigated from onboard a ship—to cut the cord so that crews can haul it onboard to repair.
Photo courtesy of Orange Marine.Following the granular details of the surveys that Orange Marine’s bathymetry vessels would have taken months before, the Rene Descartes will chug forward at about 9 miles per hour, sometimes more quickly if the cable is running down an underwater incline, sometimes more slowly if up an ascent.
Each of the ship’s movements is guided by what is known as differential GPS, a system that allows not just the ship as a whole, but its bow and stern, to occupy different sets of coordinates down to the centimeter.
These coordinates are fed into another bank of computers that guides how quickly or slowly the cable gets paid out.
At each end of a cable is a landing station, often as big as a house and usually tucked away in some unassuming seaside settlement—near, rather than in, a bustling harbor, an industry practice that keeps the cables inconspicuous and away from the hardware dragged by shipping and fishing traffic.
The fundamental purpose of the landing stations is to shoot light frequencies carrying our data down the hair-thin tendrils of fiberoptic glass that run down the center of each cable.
For this light to travel the enormous distances between landing stations and maintain its original strength, about 10,000 volts of electricity are pumped from both sides of the cable down a copper sleeve that cocoons the fiberoptic strands.
This electricity powers signal amplifiers called repeaters.
All of this AC voltage is grounded by the seafloor itself, Dillard explains.
The repeaters lie on the ocean floor along the cable at intervals of about 50 miles.
Each is a pressurized chamber that weighs about 500 pounds and creates a bulge in the cable that resembles a rat just swallowed by a snake.
Within them, little spiral tracks of erbium are charged to goose along the photons that make up our emails, newspaper subscriptions, and cat videos.
The most perilous part of any cable’s journey is through the shallows near their landing stations.
At less than about 3,000 feet of depth, the cable will take on additional armor, usually steel rods and, closer to shore, a shell of cast-iron piping.
During this stretch of the journey, a cable will ordinarily be buried.
For this purpose, the Rene Descartes, for example, has an enormous plow it can lower to dig trenches through the seabed, the cable laid in the furrow from the plow’s trailing edge.
Then, when the water eventually becomes too shallow for the ship itself to progress, the cable is floated to shore on a series of balloons, one every few feet holding the cable along the surface while a bevy of technicians guide it to its plug-in on shore.
The balloons are then snipped off, the last yards of the cable buried, and the very end wired into the network of whatever landmass is the next stop on the information superhighway.
As Tonga—or other recent failures like the
SEACOM and
EASSy cable outages around South Africa last spring—reveal, things can and do stumble over the network.
And it doesn’t always take something as catastrophic as a volcano to foul things up.
In fact, natural events like eruptions, earthquakes, or freak currents only account for about
12 percent of disconnects.
The most common emergencies that any of Orange Marine’s six globally stationed vessels respond to are cases where dragging anchors slice cables or fishing trawlers snare and sever them.
But how on earth do you locate a break along thousands of miles of cable at the bottom of the ocean? For electricians at the landing stations, it’s a relatively easy process: spread-spectrum time-domain reflectometry.
In simple terms, a landing station sends out a special electrical “ping”—much like sonar—and waits for the echo.
The so-called spread-spectrum signal means that it’s scrambled into a unique digital fingerprint that distinguishes it from other electrical noise on the cable.
When the ping bounces back, the cable operators can detect the coordinates of the break.
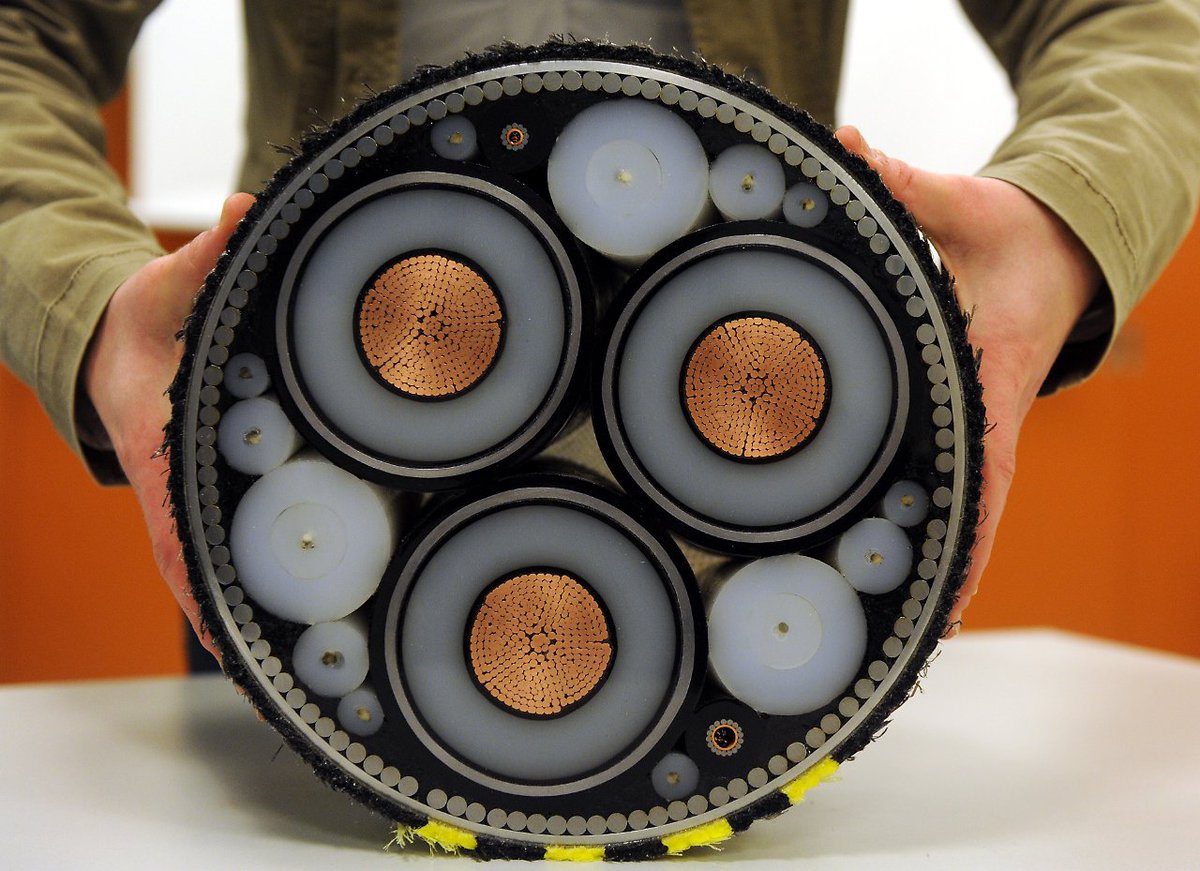
A submarine cable generally consists of a lead sheathed cable and is usually armored.
This is a cross section of a submarine power cable used to connect mainland areas or cities via water passages.
(30cm of cable costs around $400)
How do you locate a break along thousands of miles of cable at the bottom of the ocean?Arriving at the site, a repair ship again has to manage problems of slack—or rather lack of it.
If the cable has been laid to the exacting specifications of its survey, hugging the contours of the seafloor, you can’t just drop a hook and fish it out.
It’s too tight.
Instead, the technicians on the ship will lower a grapnel, which the ship will then hitch up under the cable to just slightly raise it from the ocean floor.
The ship’s ROV will be lowered to the sea floor, where it churns toward the cable with shears and cuts it off.
At this point, yet another hook can be lowered to retrieve the loose end of the cable and haul it onto deck.
This is when something like neurosurgery begins in a special workshop on deck as four cable engineers, working two at a time in 12-hour shifts, fuse a fresh length of new cable to the broken end they just hauled up from the deep.
From here, the other cut end of the cable still on the seafloor can be hoisted aboard, and the two sides can be spliced together.
All of this typically takes days.
To ensure that the newly lengthened cable doesn’t stray from its original coordinates—which are noted precisely on navigation charts the world over—the extra length that was added during the repair must be carefully folded along the contours of the original cable.
Yet even these small additions will be blasted out to the folks that make the navigation charts so the tiny bits of extra cable can be noted and, hopefully, avoided.
As humanity’s thirst for data and communication are growing, so too, must the cables.
As of five years ago, Dillard says the cables he was laying off any of Orange Marine’s six installation vessels contained 12 gossamer strands of fiberoptic cable: six carrying humanity’s queries in one direction, six in the other.
By now, he says, the cables contain as many 24 fiberoptic strands, doubling their already searing bandwidth to about 225 terabits a second.
With such capacity, one could send all of the information in the Library of Congress to Bombay in about 12 minutes.
Or, more practically, 9 million viewers in Japan and Taiwan could simultaneously stream high-definition films from a Netflix server in the U.S. on half of a single cable with no discernable lag.
“Their capacity is unimaginable,” says Dillard.
For all their hair-raising bandwidth, the retracing of the same lines woven decades ago under the seas threatens to leave countries like Tonga adrift with their single cables or single paths of data in and out.
As Panuve told me, he’d love to see new pathways of connectivity—if anyone could be persuaded to pay for them.
For the internet to be the borderless egalitarian love-in it was always sold to be, its very hardware needs to ford new routes.
For now, the imbalance shows where the money really flows.
Links :